Introduction
Platelet-rich plasma (PRP) therapy depends not just on the concentration of platelets, but also on how and when those platelets are activated to release growth factors. While many protocols include an ex vivo activation step using agents like calcium chloride or thrombin, other clinicians prefer to inject PRP in its resting form, allowing the native collagen and tissue microenvironment to trigger activation in situ.
As noted in a 2016 review (Everts et al., PMCID: PMC5031826), “the choice of strategy to activate PRP is mainly based on practical reasons rather than supported by studies on the effects of the different procedures on the final platelet releasate.” Understanding the implications of platelet activation strategy allows clinicians to tailor PRP preparations more precisely to specific clinical scenarios. This is particularly critical when selecting between activation methods appropriate for leukocyte-poor PRP (LP-PRP), often used in intraarticular applications, versus those better suited for leukocyte-rich PRP (LR-PRP), typically employed in treating muscular or tendon injuries.
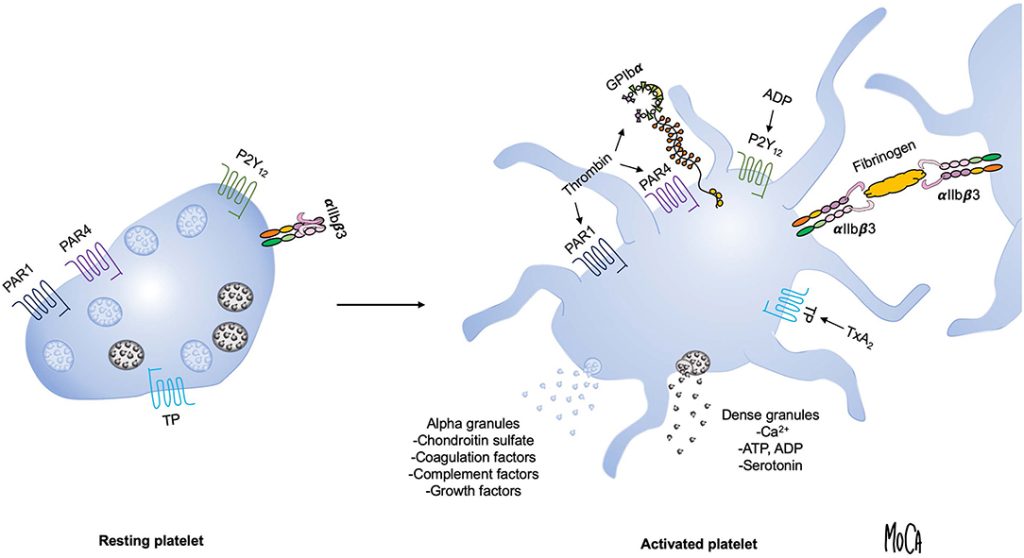
Platelet Activation Methods – Comprehensive Classification
I. Physical Activation (Mechanical + Thermal)
These methods use physical forces or temperature to induce platelet activation or lysis.
Mechanical
- Needle Shear: Forcing PRP through a narrow-gauge needle (typically 21G or smaller) generates mechanical shear stress, partially activating platelets.
- Centrifugal Shock or Manual Agitation: Abrupt deceleration or shaking causes disruption to the platelet membrane, resulting in partial activation.
- Freeze-Thaw Cycling: Complete lysis of platelets via intracellular ice formation, typically using a -80°C freeze followed by thawing at 37°C. Releases all intracellular contents and is commonly used for producing platelet lysate.
Thermal
- Mild Heating (37–42°C): Promotes natural platelet activation without rupturing membranes, potentially mimicking physiological cues.
- Freeze-Induced Lysis: Functionally identical to freeze-thaw, often grouped together in literature due to the shared mechanism of thermal and mechanical stress.
Effect: Physical methods vary in degree of activation. Freeze-thaw causes full lysis and uncontrolled growth factor release, while needle shear and thermal exposure offer partial, more controlled activation depending on intensity and duration.
II. Chemical Activation
Clinically validated agents that provide controlled and reproducible platelet activation.
- Calcium Chloride (CaCl₂): Initiates clotting cascade and platelet activation. Frequently used in leukocyte-poor PRP for intraarticular applications. Offers strong but modulated activation without excessive inflammation.
- Thrombin (Autologous or Bovine): A powerful activator that rapidly induces platelet degranulation. Preferred in surgical settings and for leukocyte-rich PRP applied to muscular or soft tissue injuries.
- Calcium Gluconate: Milder than CaCl₂, capable of forming a fibrin scaffold and activating platelets. Used in protocols where gradual release is preferred or lower procoagulant activity is desired.
- Collagen (Type I or Peptide Fragments): Binds to platelet surface receptors to mimic natural vascular injury. Less commonly used in clinical settings due to complexity and variability in source materials.
- Sodium Bicarbonate (adjunctive): Occasionally used to modulate pH or in combination with calcium to enhance fibrin matrix formation. Rarely used alone for primary activation.
Effect: Chemical activators offer precise control over platelet activation and fibrin formation. The choice of agent affects clot kinetics, inflammatory potential, and timing of growth factor release.
III. Ultrasonic (Ultrasound-Based) Activation
A non-contact, equipment-based method that activates platelets via mechanical stress from acoustic waves.
- Mechanism: Low- or high-intensity ultrasound generates cavitation and shear forces that disrupt platelet membranes and stimulate degranulation.
- Typical Parameters: Frequencies around 1 MHz, intensities of 1–2 W/cm², with exposures between 30 seconds and 2 minutes.
Advantages:
- No chemical additives
- Sterile and tunable
- Allows spatially targeted activation
Limitations:
- Requires specialized ultrasound devices
- Less commonly used in standard PRP workflows
- Potential for overheating or overactivation at high intensities
Application I: LP-PRP for Intraarticular Injections
Goals: Controlled anti-inflammatory response, sustained growth factor (GF) release, minimal leukocytes/fibrin.
Best Activator: Calcium Gluconate
- Provides a slower, more sustained calcium ion release
- Leads to prolonged, spatially targeted growth factor delivery
- Aligns with low-vascularity, slow-healing joint environments such as cartilage and synovium
- Lower inflammatory profile compared to CaCl₂ or thrombin
Alternative: Resting PRP (No Activation)
- Activated by native collagen in cartilage/synovial matrix (Everts et al., 2016)
- Promotes spatial precision of GF release at lesion site
- Preserves fluid consistency, enhancing injectability
Caveats:
- Delayed onset of therapeutic action
- Variability depending on tissue condition and collagen exposure
- Fibrin formation is limited, reducing scaffold formation unless calcium is present
Multiple Injections Benefit: Because of the inherently low vascular supply to intraarticular cartilage and synovial tissue, nutrient and growth factor delivery to these regions is naturally slow and limited. A single bolus of activated PRP may not sustain its effects long enough to meaningfully impact tissue remodeling. The use of non-activated or slowly activated PRP—such as when using calcium gluconate—aligns with a theoretical advantage for serial dosing. By delivering multiple injections over time, clinicians can maintain a therapeutic concentration of growth factors within the joint as the healing response progresses.
Supporting evidence from clinical trials confirms this:
- Dai et al., 2023: Multi-injection PRP better than HA at 3, 6, and 12 months (PMC10057845)
- Xing et al., 2024: 3 injections optimal vs. 1 or 5 (PMC11077828)
- Korpershoek et al., 2024: Improved function and pain with repeated PRP (PubMed 38392616)
Spatial Precision Explained:
- Activated PRP releases GFs systemically, risking off-target dispersion
- Resting PRP and slow-activating agents like calcium gluconate activate only at injury site, focusing therapeutic payload
- Higher GF bioavailability at the lesion, lower systemic inflammatory risk
Application II: LR-PRP for Muscular Lesion Treatment
Goals: Immediate GF release, fibrin matrix formation, immune cell engagement
Best Activator: Autologous Thrombin
- Rapid, complete activation
- Creates fibrin scaffold for adherence
- Supports acute repair, especially in tendons and muscle
Alternatives:
- CaCl₂ if slower release acceptable
- Ultrasound for non-contact partial activation
Avoid: LP-PRP and weak activators due to lack of inflammatory and structural support
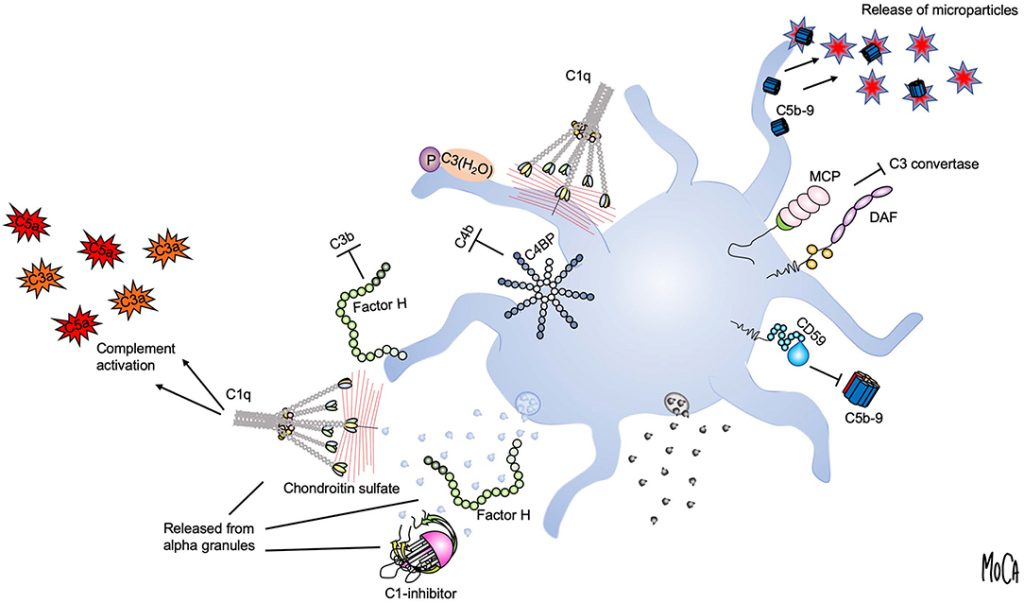
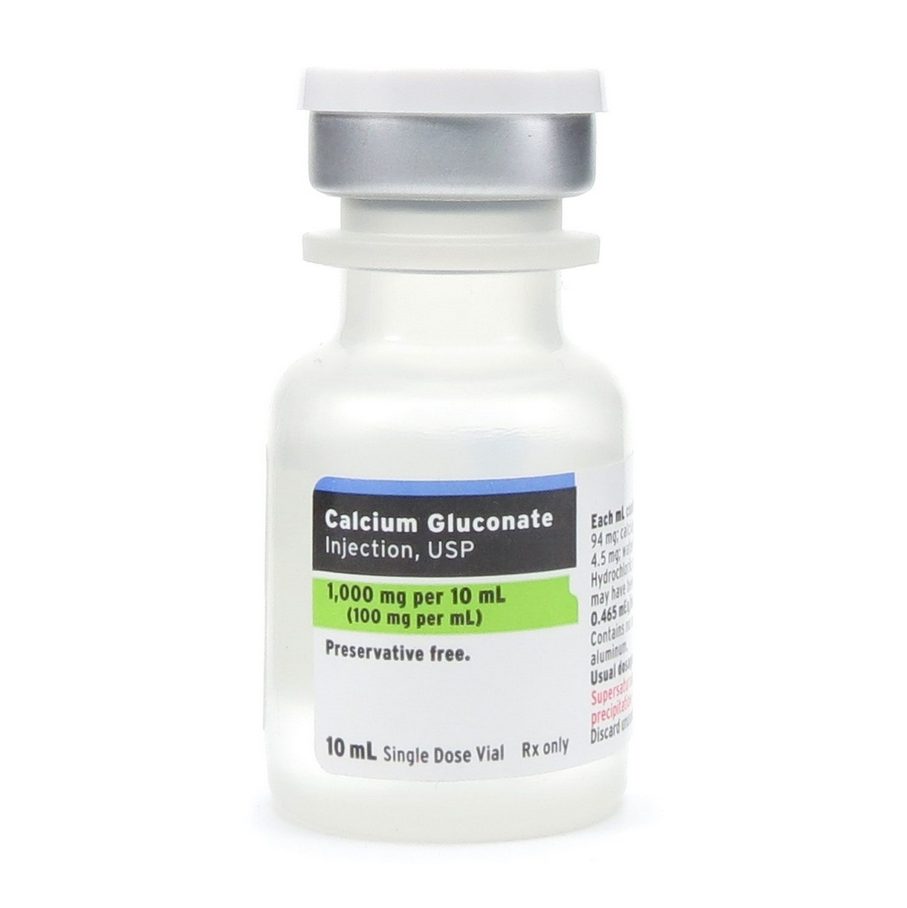
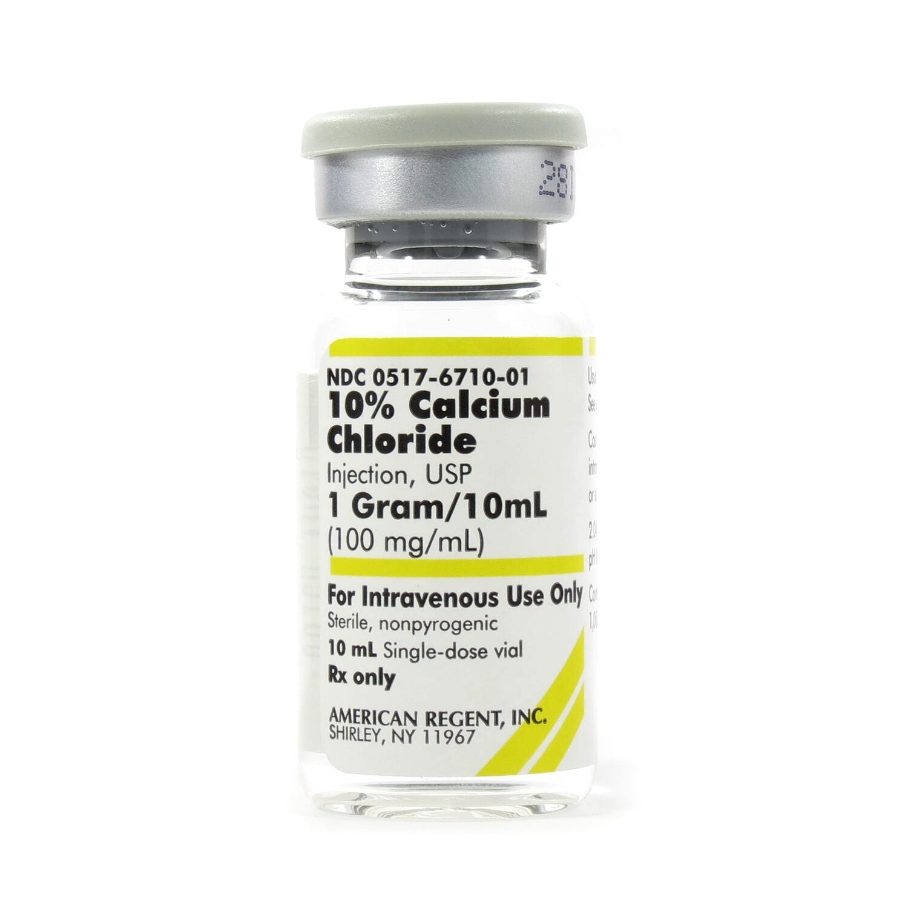
Calcium Gluconate vs. Calcium Chloride: IA Implications
Calcium Chloride:
- ~27% elemental calcium
- Fast dissociation, quick GF release
- Ideal for acute-phase, rapid activation
Calcium Gluconate:
- ~9% elemental calcium
- Slower dissociation, sustained GF release
- Less inflammatory; ideal for prolonged healing
Joint Biology Considerations:
- Cartilage and synovium are avascular and slow-healing
- Slower GF release matches extended cellular repair timelines
- Calcium gluconate better aligns with low-flow, chronic healing environments (PMC9339933)
Note on ACD-A Anticoagulant and Calcium-Based Activation
Most PRP preparation systems use ACD-A (acid citrate dextrose solution A) as the anticoagulant during blood collection. ACD-A works by chelating free calcium ions, thereby preventing clotting and spontaneous platelet activation. However, this mechanism has direct implications for subsequent chemical activation. If the citrate is not adequately neutralized, it can continue to bind calcium and inhibit the effectiveness of calcium-based activators such as calcium chloride or calcium gluconate. To achieve reliable activation, clinicians must add sufficient calcium to overcome the chelating effect of ACD-A. Failure to do so may result in incomplete platelet activation and impaired fibrin matrix formation. The specific calcium dose required should be calculated to exceed the binding capacity of citrate while accounting for the desired activation kinetics.
Conclusion: In intraarticular applications, calcium gluconate may be theoretically superior to calcium chloride due to its delayed activation kinetics, which better match the protracted biological repair processes in low-vascular joint spaces. Its use may support sustained trophic signaling over the weeks-long course of cartilage healing, particularly in multi-injection protocols.
Suggested Further Reading
For readers interested in a deeper understanding of platelet activation mechanisms and their immunological significance, we recommend the following open-access review:
- Assinger, A. (2019). Platelets and innate immunity: mechanisms and interactions. Frontiers in Immunology, 10, 1590. https://www.frontiersin.org/articles/10.3389/fimmu.2019.01590/full
References
- Everts, P. A., et al. (2016). Reviewing the mechanisms of platelet-rich plasma therapy in musculoskeletal injuries and osteoarthritis. International Journal of Molecular Sciences, 17(8), 1123. PMCID: PMC5031826.
- Dai, W. L., et al. (2023). The efficacy of platelet-rich plasma for knee osteoarthritis: A systematic review and meta-analysis. BioMed Research International, 2023. PMCID: PMC10057845.
- Xing, J., et al. (2024). Three versus five PRP injections for knee osteoarthritis: A randomized controlled trial. International Orthopaedics, 2024. PMCID: PMC11077828.
- Korpershoek, J. V., et al. (2024). Repeated intra-articular PRP injections improve knee function and reduce pain: A longitudinal cohort study. Journal of Orthopaedic Research. PubMed ID: 38392616.
- Zhou, Y., et al. (2016). Ultrasound-induced platelet activation for biomedical applications. Journal of Translational Medicine, 14, 335.
- Shen, L., et al. (2013). Optimization of ultrasound parameters for platelet activation. Ultrasound in Medicine & Biology, 39(6), 1056–1065.
- Al-Hamed, F. S., et al. (2022). Comparative evaluation of calcium chloride and calcium gluconate in PRP activation. Journal of Clinical Medicine, 11(17), 5035. PMCID: PMC9339933.
Leave a Reply